Designing with UWB: Short-Range Wireless Apps Reimagined with Performance Far Beyond Bluetooth
January 20, 2022
Story
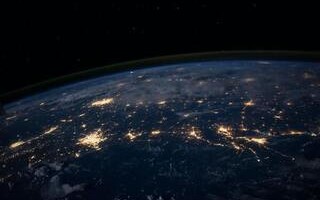
With 10x faster data throughput, 60x less latency, and 40x better energy efficiency, UWB prompts a fresh look at design considerations from payload sizing to testing/certification and antenna design.
Wireless device engineers have been hearing a lot about ultra-wideband (UWB) in recently. The high-profile launch of Apple’s UWB-based AirTags tracking and location devices earlier this year turned a lot of heads — if not for the AirTags themselves, then for the fact that Apple stealthily designed a UWB transceiver into its iPhone, where available real-estate to fit additional components is scarce.
Apple found a way, and it no doubt has bigger plans for UWB in its product portfolio going forward. Samsung and a host of automotive OEMs have likewise planted their flags on the UWB frontier, and many more will follow.
But the ranging, positioning, and automotive keyless entry applications where UWB is currently gaining traction only scratch the surface of UWB’s potential for short-range wireless connectivity. To fully appreciate UWB’s capabilities, consider its performance attributes relative to Bluetooth, our de facto short-range wireless platform since the turn of the century.
With UWB, wireless device designers can achieve huge leaps forward in performance capabilities compared to legacy Bluetooth. This will impose a modest learning curve as designers familiarize themselves with new design approaches, and Bluetooth’s ubiquity is such that many designers have been engineering Bluetooth-based wireless devices their entire professional careers.
However, UWB’s breakthrough performance benchmarks make it worthwhile. And as we contemplate UWB’s impact on device design considerations and workflow, the advantages become even more obvious.
Payload Size and Data Rate
Depending on the target application, designers leveraging UWB transceivers will likely encounter very different payload sizes and packet rates than what they’re accustomed to with Bluetooth. Consider wireless audio applications, where Bluetooth has been stifling audio quality for decades with lossy codecs that compress CD-quality audio from 1,411 kbps down to just 300 kbps data delivery.
When sizing the payload of an uncompressed, 16-bit audio signal at 48kHz sampling frequency, in stereo, UWB delivers this audio signal at approximately 2 Mbps, with 20% overhead factored in to allow for retransmission if needed. For designers targeting premium audio applications with uncompressed audio at 96kHz sampling frequency, the data rate doubles to 3.8 Mbps. This is far out of reach for Bluetooth-enabled wireless audio apps and is in fact more akin to video streaming rates with H.264 compression applied.
It’s important to pause here and consider the many conceivable high-data-rate, short-range wireless communications applications that have never been attempted due to Bluetooth’s inherent data rate limitations, shutting down considerable innovation that UWB enables.
Liberated from Wires (and Batteries)
Consider the many short-range communications apps that still rely on physical wires, with all of the size, weight, space, cost, and deployment compromises that wired comms imply. These devices can now be unplugged, and IoT/IIoT sensors provide a great example of how this could transform sensor-based applications.
Typically for IoT sensors, payloads may measure just a few bytes transmitted every few milliseconds – 10 to 100 kbps data rates may suffice. For the next generation of sensors targeted for use in autonomous vehicles and smart infrastructure – among countless other AI-guided commercial and industrial apps – sample rates like this won’t be anywhere close to enough to satisfy AI’s insatiable data demand. "Always on" availability for live telemetry will be required to access and act on the most up-to-date data available.
The onboard Bluetooth chip consumes a disproportionate 80% of the entire sensor power budget on average today, so the sensor batteries must be frequently recharged or replaced, a time- and resource-intensive process. Or, as a significant compromise, the sensor simply cannot continuously send its telemetry and needs to send bursts of data between long time intervals, defeating the "always on" paradigm. It’s for this reason that many of today’s sensor networks are still reliant on hardwired connectivity, even after all the progress made in high-performance wireless connectivity.
For the many IoT sensor applications that will continue to be serviced at 10 to 100 kbps data rates, UWB will still prove advantageous over Bluetooth. In SPARK Microsystems’ UWB implementation, 20% or less of the sensor power budget is consumed by the wireless comms chip. Depending on how designers are using their sensors, this could enable "set it and forget it" sensor installations that operate for many years before a drained battery ever becomes an issue. With so little power consumed by the UWB transceiver, this also opens the door to a future of battery-less sensors powered by nothing more than ambient indoor light, or even vibration or body heat.
The advent of battery-less, wireless sensors will likewise be a watershed moment across a range of application domains, with major implications for sensing and AI at the edge.
Testing and Certification
In the United States, the Federal Communications Commission in 2002 authorized the unlicensed use of UWB in the frequency range from 3.1 to 10.6 GHz providing UWB an advantage over narrowband, given its allocation of and support over such a large portion of radio spectrum.
Based on emissions regulations set in the 2000s, an UWB signal is defined as a signal having a spectrum larger than 500 MHz. Most countries have agreed on the maximum output power for UWB, defined as -41.3 dBm/MHz, to avoid disturbing adjacent wireless communications.
UWB testing and certification labs are accessible to help designers validate their compliance to emissions regulations, leveraging established protocols. UWB and application experts are likewise at the ready to help device designers pass these certifications with little friction.
The RF frequencies and bandwidths for Bluetooth and UWB may be different, but common principles apply when it comes to compliance testing. Designers using UWB today are already familiar with the process – the values are different, but the measurements are the same.
However, there will be a gradual need to employ testing equipment with the sensitivity to measure nanoamps. The energy efficiency optimizations applied to UWB to achieve 10x lower power than Bluetooth will require the ability to perform sub micro amp measurements, a testament to UWB’s strikingly low power consumption, considering the dramatically increased data rates that UWB enables.
Antenna Design Optimization
With today’s Bluetooth-enabled devices at 2.4 GHz, hundreds of devices could be beaming toward each other at any given time, potentially creating a lot of interference. UWB benefits from a spectrum that is relatively uncrowded at present. Innovative design techniques can help to minimize RF collisions with surrounding devices, and WiFi can comfortably coexist in this spectrum as well with WiFi 6 residing near low-end UWB spectrum. This ensures that a UWB radio can be utilized in a room full of Bluetooth and WiFi devices with little to no interference.
At the device T/R antenna layer, Bluetooth radios can transmit between 1,000 and 1,000,000 times more power than UWB radios, for which the output power must be much lower due to emissions limits. UWB transceivers must be very sensitive to signal reception, because they’re measuring signals that are very near the noise levels. So the challenge remains for designers to optimize efficient antennas to get good sensitivity.
Because the UWB frequency range is so wide, it’s also somewhat forgiving when it comes to the material properties employed at the antenna layer. With Bluetooth, a miniscule shift in material properties could easily result in a shift out of the intended frequency range; UWB can accommodate more variability in the materials, leveraging an extra hundred MHz on both sides of the signal. Likewise, the resonant frequency of BLE antennas can be easily detuned near the human body, but UWB antennas are immune to this phenomenon.
Make no mistake, antenna design is widely regarded as “witchcraft” for a reason – it calls for highly specialized design techniques. It’s commonly known that any piece of metal surrounding the antenna will impact its performance, whether it’s extraneous to the device or affixed to the device itself. Bluetooth will be a bit less sensitive to this because of its leeway to transmit more energy without running afoul of regulations. In either case, the position and configuration of the antenna within the product – be it Bluetooth or UWB-based – will remain paramount concerns to the designer.
UWB is the Future
All of the aforementioned UWB attributes distinguish UWB as the logical successor for personal area network (PAN) applications previously serviced with Bluetooth. In addition to positioning and ranging applications, UWB will set the foundation for future generations of ultra-high performance and extremely power-efficient wireless audio earphones, gaming headsets and peripherals, and countless AR/VR/MR/XR devices targeted for use in tomorrow’s metaverse.
The industry’s shift from Bluetooth to UWB-centric short-range wireless devices entails some changes in perspective when it comes to design and testing/certification processes. But the innovation that UWB can unleash delineates an upside for future wireless device designs and capabilities, liberated from wires and in some cases, batteries.