How to Improve Reliability and Efficiency of Smart Thermostats
October 21, 2022
Story
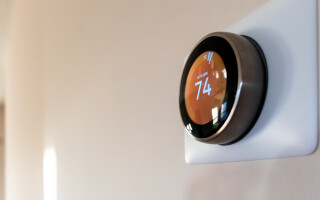
Smart thermostats have become a popular method of energy conservation for homes, office buildings, and retail facilities. The market for smart thermostats has been growing at a high CAGR of 22% since 2018. With such high growth, manufacturers can easily justify innovation in new models. Future smart thermostats will provide a wide range of information, including energy usage audits and comparative data on energy consumption before and after an HVAC system upgrade.
Smart thermostats will employ more technology, such as a wide range of IoT interfaces for control and data communication. In addition, these devices will operate from AC line power and have battery backup power to remain operational during a power outage.
To ensure a highly reliable smart thermostat, design engineers must incorporate robust components to protect the thermostat from potentially damaging external threats such as power line current overloads, voltage transients, and electrostatic discharge (ESD) strikes. In this article, designers will learn how to protect their sensitive electronic circuits from these hazards. With this information, designers will confidently be able to develop smart thermostats that can withstand hazardous transients and be highly robust and reliable.
Overview of Smart Thermostat Operation
A smart thermostat can perform a wide range of functions. As with any thermostat, the smart thermostat’s primary function is to monitor the temperature in the surrounding environment and activate the HVAC system, when necessary, to maintain the thermostat setpoint temperature. The features that make the thermostat smart include:
- communicating the HVAC system status,
- processing control information from a remote device such as a smartphone, PC, or tablet computer,
- alerting the owner or manager if an action is required.
Some smart thermostats have a battery pack for backup power if AC line power is interrupted. Figure 1 illustrates an example smart thermostat. The boxes around the image describe its functions and list recommended technologies for the protection, control, and sensing that will improve the reliability and efficiency of the smart thermostat.
Figure 1. Example of a smart thermostat
Figure 2 shows a detailed block diagram of a smart thermostat. The table to the right of the block diagram lists recommended components that protect the circuit blocks and enable efficient control and sensing. The following paragraphs describe the hazards that can damage the circuitry and the recommended protection, control, and sensing components needed for high reliability and efficiency.
Figure 2. Smart thermostat block diagram
Protecting the Smart Thermostat from Overloads, Transients, and Electrostatic Discharge
Power Input Circuit Block
The Power Input block converts the 24 VAC input from the HVAC system into the DC voltage that powers all other circuit blocks. Although the HVAC system reduces the power line AC voltage for the thermostat to 24 VAC, the 24 VAC line is still susceptible to power line overload currents and voltage transients that can pass through the HVAC system.
To protect the Power Input from sustained current overloads, use a time delay fuse to enable the fuse to withstand inrush currents caused by motors turning on and turning off. A time delay fuse will avoid nuisance shutdowns resulting from inrush currents. Miniature axial lead and surface mount fuses are available for low voltage circuits to save on precious pc board space.
A Polymeric Positive Temperature Coefficient (PPTC) resettable fuse is an alternative to a standard, one-time overload fuse. The fuse opens due to heat from an overload current; however, it resets when its temperature falls below a threshold level and does not need replacement after it has opened.
Lightning strikes on the power line and large motors inducing back EMF voltages on the power line can result in damaging transient overloads. These voltages can reach kV levels. While the HVAC system can have protection from these overloads, some of the energy can pass through the HVAC circuit to the Power Input block. Use a transient voltage suppressor (TVS) diode to clamp and absorb the residual transient voltage and its energy. TVS diodes offer:
- Ultra-fast response time of under 1 ps
- Low clamping voltages to protect sensitive semiconductors
- Ability to safely absorb peak pulse power of 400 W
- Bi-directional and uni-directional configurations
- Versions with surface mount form factors.
Figure 3 illustrates the symbols for the bi-directional and the uni-directional TVS diodes. The two diodes, shown in the bi-directional configuration, use cathode-cathode connected diodes.
Figure 3. Bi-directional TVS diodes connected cathode-cathode and a uni-directional TVS diode
Battery Management Unit
The Battery Management unit ensures that all battery cells equally power the load, and monitors the state-of-charge of the cells. Protect the semiconductor control circuitry from voltage transients and ESD with a TVS diode. Consider a TVS diode or a multilayer varistor (MLV). Either device can provide very low clamping voltages to protect low voltage semiconductors and provide ESD protection compliant to IEC 61000-4-2, the electromagnetic compatibility standard.
Designers should prevent battery overcharging and battery overtemperature conditions by using PPTC resettable fuses. Versions of PPTC fuses can be either surface-mount PC board components or directly mountable on the battery pack to quickly detect an overtemperature condition.
Input/Output Interfaces
A smart thermostat can have several wired and wireless interfaces. The interfaces are subject to hazards from the external environment, which is typically ESD strikes.
For the USB interface, use TVS diodes for ESD protection. Look for:
- A minimum safe absorption of a ±20 kV ESD either through the air or via direct human contact
- A maximum of 1 pF of capacitance for minimum distortion of transmitted and received signals
- A low clamping voltage of no more than 10 V
- A uni-directional or bi-directional diode
- Leakage current under 100 nA.
Consider a PPTC resettable fuse to protect the USB interface from current overloads. Look for a small model to save PC board space.
The Audio Input Interface outputs alarms and has voice-activated input. Protect this interface’s I/O lines with a TVS diode. Consider either a uni-directional or bi-directional diode for each line.
The Input User Interface provides a data entry panel. The Zigbee Wireless Communication Interface transmits data and receives control information from devices such as a smartphone or a tablet PC. Both interfaces require protection from an ESD strike. A TVS diode array that provides at least 10 kV of bi-directional energy absorption will protect these two interfaces safely. An alternative to the TVS diode for the Wireless Communication Interface is a polymer ESD suppressor. A polymer ESD suppressor has very low capacitance, typically around 0.25 pF, to minimize its impact on the RF signals, under 1 ns response time, and a typical leakage current of under 10 nA.
The RS-485 Wired M-Bus in a commercial smart thermostat provides data to an external system. Use an asymmetrical TVS diode array to protect the -7 V and +12 V working voltages on the RS-485’s data lines. Also, look for high ESD absorption above 20 kV. One component, as shown in Figure 4, protects both data lines.
Figure 4. Schematic of an asymmetrical TVS diode array for absorbing voltage transients and ESD on each of the RS-485 data lines
The last interface is the Display which, at a minimum, shows the temperature setting and actual room temperature. A TVS diode such as the one recommended for the Battery Management Unit or a multilayer varistor can protect the display circuitry.
Efficient Control and Sensing
Consider a latching relay driver to control power to the smart thermostat's circuit blocks. To avoid generating electromagnetic interference (EMI) during switching, look for a driver that switches when the AC line voltage passes through 0 V. Also, latching relays save on power consumption since they do not draw power in the closed-contact state.
The most critical element of the smart thermostat is the temperature sensor. Consider using a thermistor, a negative temperature coefficient (NTC) element. Thermistors offer better accuracy than other temperature sensor types and detect a wide temperature range, -55 to + 220° C. Use a hermetically sealed thermistor to obtain long-term reliability and stability. Small, surface-mount versions save PC board space and allow automated insertion.
Applicable Safety Standards for Smart Thermostats
Designers need to be aware of the standards that apply to smart thermostats, and Table 1 lists these safety standards. Failure to comply with the appropriate standards can result in failure to pass compliance certification testing, additional development costs, and delayed introduction to the market.
Table 1. Safety standards for smart thermostats
Designing in Protection and Long-Life Components Achieves High Reliability
Designers need only a small number of components to protect a smart thermostat and enhance its reliability thoroughly. It is challenging to be an expert in all aspects of electronic design, so designers can take advantage of the expertise of a manufacturer's application engineers on circuit protection and efficient control. They can help with:
- Cost-effective component selection
- Guidance on complying with the applicable safety standards
- Pre-compliance testing to help identify problems before compliance testing (service provided by some manufacturers).
Using the recommended protection, control, and sensing components will ensure a reliable smart thermostat with robust protection from external hazards while providing efficient operation.
References
- Building & Home Automation Application Guide, 2020 Littelfuse, Inc.
- Circuit Protection Products Selection Guide, 2020 Littelfuse, Inc.
- Fuseology Selection Guide, 2014 Littelfuse, Inc.
- Electronic Discharge (ESD) Protection Design Guide, 2016 Littelfuse, Inc.
- Temperature Sensor Overview: Thermistors, RTDs, Probe Assemblies, 2020 Littelfuse, Inc.
Ryan Sheahen is the Global Strategic Marketing Manager for the Electronics Business Unit. Ryan joined Littelfuse in 2011 as an inside sales specialist. He was previously the Global Product Manager for the magnetic sensing product portfolio. His current responsibilities include developing marketing collateral, managing marketing activities for new product launches, and performing marketing studies and feasibility analysis for new product ideas. Ryan earned his BS in Mechanical Engineering Technology from Purdue University.